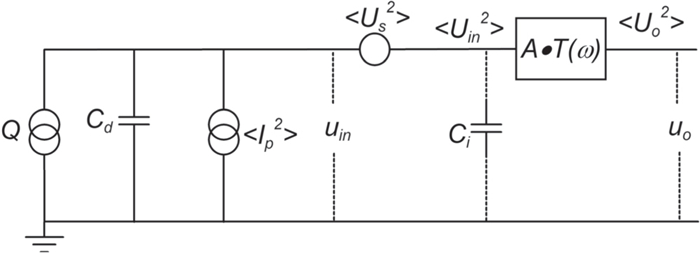
Citation: | Guoliang YUAN, Zuowei WEN, Lingfeng WEI, Jinwen ZHANG, Qingwei YANG. Neutron yield measurement system of HL-2A tokamak[J]. Plasma Science and Technology, 2022, 24(6): 064006. DOI: 10.1088/2058-6272/ac4f40 |
This research presents the development of HL-2A neutron yield measurement which includes 235U fission chamber and BF3 and 3He proportional counters. Equivalent noise formula of the radiation detection signal amplification system was derived to guide the development of the signal amplification system. Then all detectors were calibrated in situ by using the 252Cf neutron source. The neutron yield of the HL-2A during neutral beam heating was analyzed. These results indicate that the developed neutron flux diagnostic system can obtain neutron yield results under various experimental conditions of the HL-2A tokamak, and can provide information on neutron yield.
The ultimate goal of fusion energy research is to obtain the power output. The measurement of fusion power or neutron yield is basic requirement for large and medium tokamaks. There are two types of technologies: detector-based measurement and activation measurement [1–3]. This research focuses on the detector-based neutron yield measurement of HL-2A tokamak because it can provide time-resolved results.
When tokamak operates, plasma generates a mixed radiation of hard x-rays, gamma rays and neutrons. The gas ionization detector has a strong anti-interference ability against hard x-rays and gamma rays due to its small ionization cross-section, and is a good choice for neutron detection. 235U fission chamber and BF3 and 3He proportional counters are widely used in the neutron flux measurement. The principles of three types of detectors are as follows:
(a) fission chamber, the neutron reaction with the fissile material producing fission fragments and neutrons. The energy of fission fragments is up to 160 MeV.
(b) BF3 proportional counter
n+B105→L73i+α+2.792 | (1) |
(2) |
(c) 3He proportional counter
(3) |
The high-energy particles produced by the above neutron reactions generate ionization signals in the gas ionization detector. Among three types of reactions, the fission reaction releases the largest reaction energy. Therefore, the fission chamber has the best performance on the anti-interference ability of hard x-rays and gamma rays, and is primary neutron yield detector in TFTR [4, 5], JET [6], JT-60U [7], LHD [8], EAST [9, 10] and ITER [11, 12]. BF3 and 3He proportional counters can be used in lower neutron yield measurement [7, 9, 10].
In this work, we present the development of HL-2A neutron yield measurement system which is based on a 95% enriched 235U fission chamber and BF3 and 3He proportional counters, and in situ calibration results. Section 2 starts from the theoretical derivation of the equivalent noise, describes the development of the preamplifier and the results of detectors test, and finally introduces the arrangement of neutron detectors. Section 3 gives the in situ calibration work and the HL-2A experimental results, and followed by a summary and conclusion in section 4.
In previous work [13], we have developed the neutron flux detector with flat energy response and tested it on the mono-energy neutron source. However, it was found difficult to suppress the noise of the 235U fission chamber during the development process. Researches [14–16] on the fission chamber did not provide detailed information in this field. It is necessary to analyze the noise of the fission chamber. Some researchers [17] deduced equivalent noise charge (ENC) for typical detector and amplifier system, however it can be seen from the derivation here that the derivation process needs further improvement.
For a typical signal amplification system, as shown in figure 1, the transfer function of amplifier is A·T(ω), in which A is gain independent frequency and T(ω) is frequency response. The noise is equivalent to the input noise source of the spectral density:
(4) |
where a and Af are white and 1/f term of series source, respectively, and b and Bf are white and f term of parallel source, respectively. The white series noise is mainly due to the thermal noise affecting the drain current of the preamplifier input field effect transistor (FET). The 1/f series noise arises mainly from the 1/f component of the drain(collector) current noise of the preamplifier input transistor [18]. Parallel noise arises from the detector leakage current, the first input current (base current or FET gate current) and thermal noise of detector bias resistors. The spectral densities considered here are mathematical, defined on the (-∞, +∞) frequent range.
The noise voltage at input can be written as:
(5) |
where CT is total input capacitance including detector capacitance Cd and input capacitance of preamplifier Ci. Therefore, the output noise voltage can be calculated by
(6) |
Substituting equations (4) and (5) into equation (6), we get:
(7) |
T(ω) is a function with the dimension of a frequent. The output noise voltage provided by equation (7) can be written in a more significant way in dimensionless. For a certain amplification system, τ is characteristic time-constant or the peaking time, standard deviation or full-width at half maximum, or base width. Putting x/τ for ω in the integrals of equation (7), x is dimensionless parameter, and the output noise becomes:
(8) |
When a signal with a charge of Q is generated on the detector, the voltage at the input terminal is
(9) |
The radiation detection signal amplification system amplifies the input signal. Within a certain frequency range, the amplification factor is independent of frequency. The maximum signal voltage at the output terminal can be written as follows:
(10) |
where k is a constant independent of frequency. Let
(11) |
By putting:
(12a) |
(12b) |
(12c) |
the final form of squared equivalent noise charge becomes:
(13) |
The equivalent noise charge is therefore represented through the total capacitance CT, the noise parameter a, b, and Af, the time-constant τ and three shape factors A1, A2, and A3 of the amplification system. Table 1 provides calculated values of shape factors in various amplification systems including the widely-used CR n -RC n system.
Description |
|
k | A1 | A2 | A3 | |
1 | Indefinite cusp |
|
1 | 1 | 0.64 | 1 |
2a | Truncated cusp |
|
0.63 | 2.16 | 0.77 | 0.51 |
2b | Truncated cusp |
|
0.87 | 1.31 | 0.71 | 0.78 |
2c | Truncated cusp |
|
0.95 | 1.10 a | 0.67 | 0.91 |
3 | Triangular |
|
1 | 2 | 0.88 | 0.67 |
4 | Trapezoidal |
|
1 | 2 | 1.38 | 1.67 |
5 | Piecewise parabolic |
|
1 | 2.67 | 1.15 | 0.77 |
6 | Sinusoidal lobe |
|
1 | 2.47 | 1.22 | 1 |
7a | CR-RC |
|
0.37 | 1.85 | 1.18 | 1.85 |
7b | CR-RC2 |
|
0.27 | 0.85 | 1.09 | 2.56 |
7c | CR-RC3 |
|
0.22 | 0.62 | 1.06 | 3.11 |
7d | CR-RC4 |
|
0.20 | 0.51 | 1.04 | 3.58 |
8a | CR2-RC |
|
0.2306 | 3.53 | 1.5 | 1.18 |
8b | CR2-RC2 |
|
0.13 | 1.83 | 1.56 | 1.83 |
8c | CR2-RC3 |
|
0.09 | 1.44 | 1.63 | 2.40 |
8d | CR2-RC4 |
|
0.069 | 1.25 | 1.69 | 2.91 |
9 | Gaussian |
|
1 | 0.89 | 1 | 1.77 |
10 | Clipped approximate integrator |
|
0.39 | 2.54 | 0.85 | 0.69 a |
11 | Bipolar triangular |
|
1 | 4 | 2 | 1.33 |
Corrected Gatti's results [17]. |
The fission chamber is a type of gas ionization chamber which is based on collection of the charges created by direct ionization within the gas. Its electrode is coated with fissile materials. Fissile materials are radioactive and mainly emit alpha particles with MeV energy, which causes additional parallel noise. In order to reduce the impact of parallel noise, time-constant τ of signal amplification system should be small according to equation (13). The small τ is also benefit to increase the counting rate.
The detector capacitance Cd of fission chamber is very large. Then it can be concluded from equation (13) that the ENC mainly comes from the series white noise. It is necessary to decrease the white series noise figure which is mainly due to the channel resistance noise of FET. With reference to the results of other researchers [19, 20], the FET with low white series noise figure is used as the input stage, and the commercial operational amplifier is used as the amplifying stage and output stage, thus a compact charge preamplifier has been developed [21].
Then the preamplifier and the 235U fission chamber detector were tested together. In the test, a 5-meter RG-59 cable, which is low capacitance (52 pf/m), is used to connect the detector and the preamplifier. The total input capacitance reached 810 pF. The output of the preamplifier is shaped by a specially designed CR-RC amplifier and sent to a multi-channel analyzer (Canberra Multiport Ⅱ) in order to observe the pulse height spectrum. The noise amplitude spectrum is obtained when no bias voltage is applied to the 235U fission chamber. When the 235U fission chamber is biased by high voltage, the alpha signal amplitude spectrum is obtained. The acquisition time of the noise spectrum and the alpha signal amplitude spectrum is one minute. The neutron signal amplitude spectrum was obtained by placing the 235U fission chamber in a 252Cf neutron source for 72 h. The amplitude spectra of noise, α and neutron signals obtained at different time-constant are shown in figure 2 [21]. The value of the pulse height is obtained by converting the charge into energy by the argon ionization energy (26.4 eV). The three time-constants of the upper, middle and lower graphs are respectively 0.1 μs, 1 μs and 10 μs. From these amplitude spectrums, it can be found that when the time-constant is 1 μs, the noise is the lowest. It is because that the 10 μs time-constant case increases the parallel noise contribution, and 0.1 μs time-constant case increases the series noise contribution. Figure 2 also shows that alpha signal is the lowest in the 0.1 μs time-constant case. It is considered that the short time-constant decreases the probability of alpha signal accumulation. In the HL-2A experiment, the time-constant of 235U fission chamber system is set to 0.1 μs.
Proportional counter is a type of gas ionization detector which relies on the phenomenon of gas multiplication to amplify the charge produced by the primary ion pairs within the gas. Therefore, pulses are larger than those from ionization chambers under the same conditions, and the effect of noises is slightly.
Compared with the 3He proportional counter, the BF3 proportional counter has a larger reaction energy, so it has a better performance in anti-interference ability against hard x-rays and gamma rays. In addition, the 3He proportional counter can obtain greater sensitivity and can be used for lower neutron flux measurements. Three proportional counters with different sensitivities are installed on the HL-2A, and their parameters are shown in table 2.
Detector indicator | Manufacture | Parameter | Moderator | Major radius (m) |
1 g 235U fission chamber | Beijing Nuclear Instruments Factory, China | Φ50 mm×500 mm | Polyethylene, 50 mm Cadmium, 1 mm | 11.10 |
0.005 mol BF3 proportional counter | Centronic LTD, UK | Φ25 mm×500 mm, 0.5 atm | Polyethylene, 62 mm | 6.58 |
0.02 mol 3He proportional counter | Centronic LTD, UK | Φ25 mm×500 mm, 2 atm | Polyethylene, 62 mm | 6.58 |
0.1 mol 3He proportional counter | Wuhan Newradar Special Gas Co., LTD, China | Φ57 mm×230 mm, 4 atm | Polyethylene, 71 mm | 4.01 |
The capacitance of the proportional counter is small due to and its very thin anode. Therefore, series noise is not serious. The signal amplification of the proportional counter is simple. It should be noted that the time-constant of the signal amplification system cannot be too small to avoid ballistic loss.
Three proportional counters were tested in a polyethylene moderator housing irradiated by a neutron source. The same preamplifier, amplifier and MCA as in the above test were used. The pulse amplitude spectrum is shown in figure 3. The top one is BF3 counter, and shows two branch reactions. The middle one is 3He counter. The full energy peak and the so-called wall effect can be clearly seen. The bottom one is 3He counter, and its wall effect is very slight due to its large radius and high gas pressure.
All detectors are placed inside polyethylene moderator housings to achieve flat energy response [13]. In order to increase the measurement range, the most sensitive detector is placed at the position closest to the HL-2A tokamak, and the least sensitive detector is placed at the farthest position from the HL-2A tokamak, as shown in figure 4. All detectors are placed at the mid-plane of HL-2A tokamak. 1 g 235U represents the 235U fission chamber which is arranged between the toroidal field coils (TFC) 10 and 11; the 0.005 mol BF3 and 0.02 mol 3He proportional counters are arranged between TFC 9 and 10; the 0.1 mol 3He proportional counter is arranged near to TFC 4.
The absolute detection efficiency of the neutron flux monitor needs to be calibrated so that the output of the detector can provide the information of the neutron yield, or the fusion power. Large devices such as JET [1, 6], JT-60U [7], TFTR [4, 5, 22] and LHD [23] have carried out in situ neutron calibration, and obtained good experimental results.
In the spring of 2021, the detection efficiency of the neutron flux detectors was calibrated using a 252Cf neutron source with an intensity of 2.2×107 neutrons per second. The 252Cf neutron source is always located at magnetic axis. With reference to the support plate in the vacuum vessel, 16 homogeneous points are calibrated in the toroidal direction. The amplifier outputs of four detectors are recorded and processed online by Keysight M3100 digitizer (FPGA programmable, 14 Bits, 100 MSa/s).
The detection efficiency varies with toroidal angle between detectors and neutron source, as shown in figure 5. When the detector and the neutron source are at the same toroidal angle, the detection efficiency is high, and it decreases as the toroidal angle increases. The ratio of maximum efficiency to minimum one for a detector is in the range of 10–100. It is shown that the detection of neutrons is localized. In order to obtain reliable neutron yield results, it is necessary to arrange multiple detectors in the toroidal direction. The detector efficiency is calculated directly by averaging the efficiency of each detector, as shown in table 3.
Detector | Detection efficiency (Detector count/Neutron source) |
1 g 235U fission chamber | 2.86×10-8 |
0.005 mol BF3 proportional counter | 2.87×10-6 |
0.02 mol 3He proportional counter | 1.13×10-6 |
0.1 mol 3He proportional counter | 1.64×10-5 |
The charge collection time of the 235U fission chamber, 3He and BF3 proportional counters are different, and the measured values are 0.1 μs, 2 μs and 0.2 μs, respectively. Therefore, it can be assumed that the maximum counting rates in the counting mode are 500, 10 and 100 kHz. The calculated measurement range of the four detectors is shown in figure 6. It can be seen that the four detectors can cover a total neutron yield range of approximately 7 orders of magnitude from 6×106 to 2×1013 n s-1.
We revisited the results of HL-2A 37012 experiments in 2019. As shown in figure 7, the plasma current is about 180 kA, the average electron density of the center chord is (2–2.5)×1019 m-3, the neutral beam heating power is 1.4–1.5 MW, and the maximum neutron yield measured by 235U fission chamber is 7×1012 n s-1.
This work presents the neutron yield measurement system of HL-2A tokamak which is based on the gas ionization detectors, including 235U fission chamber for high neutron yield measurement and BF3 and 3He proportional counters for low neutron yield measurement. All detectors were in situ calibrated using 252Cf neutron source. The HL-2A experiment in 2019 was analyzed based on the calibration results. When HL-2A is heated by the 1.5 MW neutral beam injection, the maximum neutron yield reaches to 7×1012 n s-1.
Since the detection of the neutron flux detector is localized, further work is to develop multiple fission chamber neutron detectors symmetrically distributed in the toroidal direction, and proportional counter detector will be used to study the HL-2A Ohmic heating experiment.
This work was partially supported by the Science & Technology Department of Sichuan Province in China (No. 2021YFSY0018), National Natural Science Foundation of China (No. 11675049).
The authors would like to thank Dr. Luan Chunhong for the English language review.
[1] |
Bertalot L et al 1999 Rev. Sci. Instrum. 70 1137 doi: 10.1063/1.1149332
|
[2] |
Pu N et al 2017 Rev. Sci. Instrum. 88 113302 doi: 10.1063/1.5009475
|
[3] |
Springham S V et al 2021 Nucl. Instrum. Methods Phys. Res. A 988 164830 doi: 10.1016/j.nima.2020.164830
|
[4] |
Hendel H W et al 1990 Rev. Sci. Instrum. 61 1900 doi: 10.1063/1.1141115
|
[5] |
Barnes C W et al 1990 Rev. Sci. Instrum. 61 31511 doi: 10.1063/1.1141671
|
[6] |
Jarvis O N et al 1990 Rev. Sci. Instrum. 61 3172 doi: 10.1063/1.1141677
|
[7] |
Nishitani T et al 1992 Rev. Sci. Instrum. 63 5270 doi: 10.1063/1.1143439
|
[8] |
Isobe M et al 2018 IEEE Trans. Plasma Sci. 46 2050 doi: 10.1109/TPS.2018.2836987
|
[9] |
Zhong G Q et al 2011 Plasma Sci. Technol. 13 162 doi: 10.1088/1009-0630/13/2/07
|
[10] |
Zhong G Q et al 2016 Plasma Phys. Control. Fusion 58 075013 doi: 10.1088/0741-3335/58/7/075013
|
[11] |
Bertalot L et al 2016 IEEE Trans. Nucl. Sci. 63 1682 doi: 10.1109/TNS.2016.2553125
|
[12] |
Bertalot L et al 2019 J. Fusion Energy 38 283 doi: 10.1007/s10894-019-00220-w
|
[13] |
Yuan G L et al 2014 Plasma Sci. Technol. 16 168 doi: 10.1088/1009-0630/16/2/14
|
[14] |
Vermeeren L et al 2011 IEEE Trans. Nucl. Sci. 58 362 doi: 10.1109/TNS.2011.2113356
|
[15] |
Elter Z et al 2015 Nucl. Instrum. Methods Phys. Res. A 774 60 doi: 10.1016/j.nima.2014.11.065
|
[16] |
Alferov V P et al 2018 IEEE Trans. Nucl. Sci. 65 2421 doi: 10.1109/TNS.2018.2860986
|
[17] |
Gatti E et al 1990 Nucl. Instrum. Methods Phys. Res. A 297 467 doi: 10.1016/0168-9002(90)91331-5
|
[18] |
Bertuccio G, Pullia A and De Geronimo G 1996 Nucl. Instrum. Methods Phys. Res. A 380 301 doi: 10.1016/S0168-9002(96)00474-3
|
[19] |
Fabris L, Madden N W and Yaver H 1999 Nucl. Instrum. Methods Phys. Res. A 424 545 doi: 10.1016/S0168-9002(98)01371-0
|
[20] |
Gál J et al 1995 Nucl. Instrum. Methods Phys. Res. A 366 145 doi: 10.1016/0168-9002(95)00550-1
|
[21] |
Yuan G L et al 2020 Nucl. Instrum. Methods Phys. Res. A 968 163977 doi: 10.1016/j.nima.2020.163977
|
[22] |
Nieschmidt E B et al 1988 Rev. Sci. Instrum. 59 1715 doi: 10.1063/1.1140143
|
[23] |
Nishitani T et al 2018 Fusion Eng. Des. 136 210 doi: 10.1016/j.fusengdes.2018.01.053
|
[1] | Xiang ZHOU (周翔), Zihao LIU (刘子豪), Chao CHEN (陈超), Renjie ZHU (朱仁杰), Li ZHAO (赵丽), Lingfeng WEI (魏凌峰), Zejie YIN (阴泽杰). A novel digital neutron flux monitor for international thermonuclear experimental reactor[J]. Plasma Science and Technology, 2018, 20(6): 65603-065603. DOI: 10.1088/2058-6272/aab300 |
[2] | Yadong HUANG (黄亚冬), Benmou ZHOU (周本谋). Active control of noise amplification in the flow over a square leading-edge flat plate utilizing DBD plasma actuator[J]. Plasma Science and Technology, 2018, 20(5): 54021-054021. DOI: 10.1088/2058-6272/aab5bb |
[3] | Chen YUAN (袁晨), Jun WU (吴军), Zejie YIN (阴泽杰). A digital wide range neutron flux measuring system for HL-2A[J]. Plasma Science and Technology, 2017, 19(8): 84004-084004. DOI: 10.1088/2058-6272/aa6bf1 |
[4] | Chunyu JIANG (蒋春雨), Jing CAO (曹靖), Zihao LIU (刘子豪), Xiaofei JIANG (蒋小菲), Xianying SONG (宋先瑛), Yipo ZHANG (张轶泼), Zejie YIN (阴泽杰). Extended Bonner sphere spectrometer for dynamic neutron spectrum on HL-2A[J]. Plasma Science and Technology, 2017, 19(7): 75601-075601. DOI: 10.1088/2058-6272/aa667a |
[5] | Zhenyu WANG (王振宇), Binhao JIANG (江滨浩), Yuming YAN (严禹明), Hailong ZHAO (赵海龙), N A STROKIN. Spatial charge and compensation method in a whirler[J]. Plasma Science and Technology, 2017, 19(5): 55507-055507. DOI: 10.1088/2058-6272/aa59f4 |
[6] | YUAN Guoliang(袁国梁), YANG Qingwei(杨青巍), YANG Jinwei(杨进蔚), SONG Xianying(宋先瑛), LI Xu(李旭), WU Huajian(吴华剑), WANG Zhiqiang(王志强). Fusion Neutron Flux Detector for the ITER[J]. Plasma Science and Technology, 2014, 16(2): 168-171. DOI: 10.1088/1009-0630/16/2/14 |
[7] | XIE Xufei (谢旭飞), YUAN Xi(袁熙), ZHANG Xing (张兴), FAN Tieshuan (樊铁栓), HEN Jinxiang(陈金象), LI Xiangqing(李湘庆). Calibration and Unfolding of the Pulse Height Spectra of Liquid Scintillator-Based Neutron Detectors using Photon Sources[J]. Plasma Science and Technology, 2012, 14(6): 553-557. DOI: 10.1088/1009-0630/14/6/27 |
[8] | ZHENG Na (郑娜), ZHONG Chunlai (钟春来), FAN Tieshuan(樊铁栓). The Calculation of Prompt Fission Neutron from 233U(n, f) Reaction by Multi-Modal Los Alamos Model[J]. Plasma Science and Technology, 2012, 14(6): 521-525. DOI: 10.1088/1009-0630/14/6/19 |
[9] | YANG Zaihong (杨再宏), YOU Haibo (游海波), XIAO Jun(肖军), YE Yanlin (叶沿林). Performance Calibration using Cosmic Rays for the Multi-neutron Correlation Spectrometer[J]. Plasma Science and Technology, 2012, 14(6): 464-468. DOI: 10.1088/1009-0630/14/6/06 |
[10] | SONG Yushou (宋玉收), LIU Huilan (刘辉兰), XI Yinyin (席印印), YAN Qiang(颜强), HU Bitao(胡碧涛). The Background Simulation of a Fission Fragment Chamber in the Experiment of 209Bi(e, e’K+)209ΛPb[J]. Plasma Science and Technology, 2012, 14(5): 415-418. DOI: 10.1088/1009-0630/14/5/18 |
1. | Shen, H.-Y., Zhang, J.-L., Zhang, J. et al. FPGA implementation of 500-MHz high-count-rate high-time-resolution real-time digital neutron-gamma discrimination for fast liquid detectors. Nuclear Science and Techniques, 2024, 35(8): 136. DOI:10.1007/s41365-024-01441-1 |
2. | Wen, Z., Yuan, G., Feng, L. et al. Development of a neutron yield measurement system utilizing BF3 detectors on the HL-3 tokamak. Journal of Instrumentation, 2024, 19(2): T02016. DOI:10.1088/1748-0221/19/02/T02016 |
3. | Luo, Y., Zang, L., Qu, Y. et al. A simulation study of a collimating shield structure in a vertical neutron camera on HL-3. Fusion Engineering and Design, 2024. DOI:10.1016/j.fusengdes.2023.114086 |
4. | Zhang, J., Zhang, Y., Chen, W. et al. Investigation of energetic ion losses induced by long-lived saturated internal mode with energetic particle diagnostics in the HL-2A tokamak. Nuclear Fusion, 2023, 63(8): 086014. DOI:10.1088/1741-4326/acdca5 |
5. | Zhang, J., Zhang, Y., Zhang, J. et al. Development of a high-temporal resolution neutron flux measurement system for the HL-2M tokamak. Journal of Instrumentation, 2022, 17(7): P07027. DOI:10.1088/1748-0221/17/07/P07027 |
6. | Zhou, J., Zhang, J., Zhang, J. et al. Readout Electronics for Fast Neutron Flux Measurement with High Time Resolution on HL-2M Tokamak. Fusion Science and Technology, 2022, 78(7): 588-594. DOI:10.1080/15361055.2022.2090785 |
Description |
|
k | A1 | A2 | A3 | |
1 | Indefinite cusp |
|
1 | 1 | 0.64 | 1 |
2a | Truncated cusp |
|
0.63 | 2.16 | 0.77 | 0.51 |
2b | Truncated cusp |
|
0.87 | 1.31 | 0.71 | 0.78 |
2c | Truncated cusp |
|
0.95 | 1.10 a | 0.67 | 0.91 |
3 | Triangular |
|
1 | 2 | 0.88 | 0.67 |
4 | Trapezoidal |
|
1 | 2 | 1.38 | 1.67 |
5 | Piecewise parabolic |
|
1 | 2.67 | 1.15 | 0.77 |
6 | Sinusoidal lobe |
|
1 | 2.47 | 1.22 | 1 |
7a | CR-RC |
|
0.37 | 1.85 | 1.18 | 1.85 |
7b | CR-RC2 |
|
0.27 | 0.85 | 1.09 | 2.56 |
7c | CR-RC3 |
|
0.22 | 0.62 | 1.06 | 3.11 |
7d | CR-RC4 |
|
0.20 | 0.51 | 1.04 | 3.58 |
8a | CR2-RC |
|
0.2306 | 3.53 | 1.5 | 1.18 |
8b | CR2-RC2 |
|
0.13 | 1.83 | 1.56 | 1.83 |
8c | CR2-RC3 |
|
0.09 | 1.44 | 1.63 | 2.40 |
8d | CR2-RC4 |
|
0.069 | 1.25 | 1.69 | 2.91 |
9 | Gaussian |
|
1 | 0.89 | 1 | 1.77 |
10 | Clipped approximate integrator |
|
0.39 | 2.54 | 0.85 | 0.69 a |
11 | Bipolar triangular |
|
1 | 4 | 2 | 1.33 |
Corrected Gatti's results [17]. |
Detector indicator | Manufacture | Parameter | Moderator | Major radius (m) |
1 g 235U fission chamber | Beijing Nuclear Instruments Factory, China | Φ50 mm×500 mm | Polyethylene, 50 mm Cadmium, 1 mm | 11.10 |
0.005 mol BF3 proportional counter | Centronic LTD, UK | Φ25 mm×500 mm, 0.5 atm | Polyethylene, 62 mm | 6.58 |
0.02 mol 3He proportional counter | Centronic LTD, UK | Φ25 mm×500 mm, 2 atm | Polyethylene, 62 mm | 6.58 |
0.1 mol 3He proportional counter | Wuhan Newradar Special Gas Co., LTD, China | Φ57 mm×230 mm, 4 atm | Polyethylene, 71 mm | 4.01 |
Detector | Detection efficiency (Detector count/Neutron source) |
1 g 235U fission chamber | 2.86×10-8 |
0.005 mol BF3 proportional counter | 2.87×10-6 |
0.02 mol 3He proportional counter | 1.13×10-6 |
0.1 mol 3He proportional counter | 1.64×10-5 |