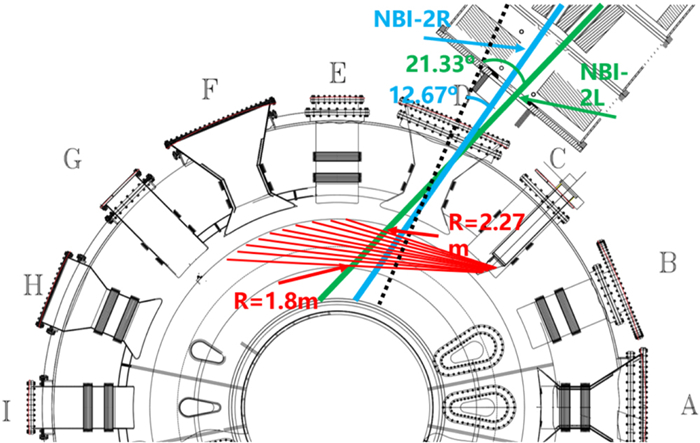
Citation: | Yichao LI, Jia FU, Yao HUANG, Jinping QIAN, Ang TI, Cheonho BAE, Shengyu FU, Jiankang LI, Yongqi GU, Zhengping LUO, Jinseok KO, Yongqing WEI, Dongmei LIU, Bingjia XIAO, Bo LYU, Xianzu GONG, Baonian WAN. Development of an upgraded motional Stark effect diagnostic system on EAST tokamak[J]. Plasma Science and Technology, 2023, 25(4): 045101. DOI: 10.1088/2058-6272/ac9b9e |
A new multi-channel motional Stark effect (MSE) diagnostic system has been developed on the upgraded EAST tokamak, which was installed on the port C to observe a tangential neutral beam. A telecentric imaging lens was deployed to ensure uniform illumination from the core to the boundary. A square fiber head which contained 23 fiber bundles was mounted to this imaging lens; each fiber bundle contained 19 fibers and two of them were assigned to CXRS and BES spectrometer, respectively. The angle tuning method was used for matching the Doppler shift of the σ component's wavelength which was caused by the beam voltage. At the present stage, the MSE system only contains ten channels that would be extended to 23 channels in the future, covering a measurement range from R = 1.8 to R = 2.27 m with a temporal resolution of 10 ms and a spatial resolution of 3 cm. The polarization angle-constrained q profiles and current density profiles were reconstructed with EFIT equilibrium reconstructions. In the sawtooth discharges, the q = 1 surface position was validated by the ECE signals, which further verified the rationality of the MSE measurement.
The motional Stark effect (MSE) diagnostic is a powerful tool to measure the current density distributions in tokamaks. Since Levinton developed the MSE diagnostic system on the PBX-M device and obtained the current density profiles for the first time in 1989, it has been developed on many tokamaks, including DIII-D, JET, TFTR, JT-60U, KSTAR, Alcator C-Mod and HL-2A, etc [1–9]. Even the low magnetic field devices such as MAST and NSTX also developed the MSE diagnostic although their low magnetic fields make it more challenging [10, 11].
In principle, when a high-speed neutral atom crosses the magnetic field line, it will be affected by the Lorentz force, which is equivalent to the effect of an electric field, thus causing the Stark energy level splitting. The emitted spectra contain σ components and π components, which are perpendicular and parallel to the Lorentz electric field, respectively. The MSE diagnostics measure the polarization direction of the Stark splitting spectra to obtain the internal magnetic field direction (i.e. pitch angle) in tokamaks, which are used in equilibrium reconstruction code to obtain more accurate plasma current density profiles and safety factor information. Recently, a multi-channel MSE diagnostic system based on photoelastic modulators (PEM) is developed and installed on EAST to measure the plasma current density distributions.
This paper gives a general introduction to the MSE diagnostic on EAST. Section 2 introduces the optical layout of the MSE, section 3 introduces the filter coupler, and section 4 describes the electronic part system. Finally, the preliminary results of the MSE are obtained and discussed.
Figure 1 shows the neutral beam configuration and the optical layout of the multi-channel MSE diagnostic on EAST. The 2nd neutral beam injection (NBI) device was transferred to the port D in the recent EAST upgrade, contained two beamlines (NBI-2R and NBI-2L), and the angle with respect to the normal direction of the port D is 12.67 degrees and 21.33 degrees, respectively [12]. The more tangential beamline (NBI-2L) was selected as the observation target because of better spatial resolution. When viewing from the port C, the radial cross-section between the sightline and the neutral beam is relatively small, the equivalent spatial resolution ranges from 0.9 to 2.3 cm according to the simulation results, which meets the current distribution demand [13]. The MSE periscope installed in the port C is illustrated in figure 1, which consists of a cover glass, a mirror, a sealing glass, a dual-PEM system, a polarizer, and a collecting lens. The mechanical design of the periscope is shown in figure 2(a). This periscope needs to preserve the polarization state of the incident light for PEM-based MSE diagnostic. To achieve this goal, the dielectric film mirror was selected to ensure the reflectivity ratio between the p and s polarizations close to unity at the incidence angle. The S-TIH6 material with a negligible Verdet constant was used to manufacture the collecting lens, which can greatly decrease the Faraday rotation caused by the magnetic field.
Figure 2(b) shows the light path of the periscope, where the light is reflected by the front mirror onto the sealing window. The object distance is 1933 mm, and the angle between the optical axis and the normal direction of the mirror is 63 degrees. The optical parameters of the periscope are shown in table 1. Limited by the size of the port volume and beam viewing aperture, only the Series II PEMs (II/FS 42–47 systems) from Hinds Instruments, Inc. can be installed in the narrow port plunge, the clear aperture of the dual-PEM is about 50 mm. To maximize the use of the dual-PEM aperture, a little bigger diameter diaphragm is placed in front of the dual PEMs. A telecentric imaging lens is adopted to guarantee uniform illumination. The focal length is 112 mm, the image numerical aperture is designed a little large than 0.22 for full-filled the numerical aperture of the silica fiber, and the total magnification of the periscope is 1/17.01.
Item | Value |
Clear aperture | 50 (mm) |
Focal length | 112 (mm) |
Objective distance | 1933 (mm) |
Object numerical aperture | 0.012 93 |
Image numerical aperture | 0.22 |
Magnification | 1/17.01 |
Optical fibers are used to deliver modulated light to the laboratory. The fiber bundles are divided into two segments. The first segment is 4 meter short fiber bundles, one end is packaged together and connected to the periscope, and the other end is dispersed and attached SMA905 connector for every single fiber. As shown in figure 3(b), there are 23 channels in the packaged end, each channel contains 19 fibers and is arranged in a double-row array, two of them are selected for CXRS and BES diagnostic respectively as shown in figure 3(a). The fiber NA is 0.22, with a core diameter of 400 μm and a cladding diameter of 470 μm. An important part of this design is that the layout of the optical fibers adopts the inclined fiber array. Due to the fact that the angle between the object plane and the NBI direction is about 35.8 degrees (figure 2(b)), the image plane needs to be tilted by an angle to adjust the object plane to coincide with the neutral beam position. Figure 3(d) shows the layout of the inclined fiber array, the fiber array is inclined by an angle of 2.1745 degrees to offset the inclination of the object plane.
The second segment is 65 m long fiber bundles. At present, only ten bundles were equipment which would be extended to 23 channels in the further. Each bundle contains 19 fibers, one end is dispersed and attached SMA 905 connector for easily connecting to the short fiber bundles. The other end is packaged as a circle, as shown in figure 3(c), this helps reduce the filter coupling angle.
The interested wavelength range (center wavelength of the σ component) is selected by the narrow bandpass filter. Based on the previous simulations, the full width at half maximum (FWHM) of the filter passing band should be 0.4 nm in order to obtain a high signal-to-noise ratio and strong signal at the same time [13]. The manufacturer of the filter is ALLUXA corporation, and the FWHM of the filter is 0.28 ± 0.1 nm, due to assembly error, the test result is about 0.4 nm. Because the center wavelength of the beam emission spectrum is different from the core to the boundary, the center wavelength of the filter comes in several different specifications ((658.66 ± 0.2) to (659.38 ± 0.2) nm). The σ component's wavelength depends on the beam voltage and the angle between the sightline and the beamline. When the beam voltage changes from 50 to 55 kV, the central wavelength of the emission spectra in the core would change by about 0.15 nm. So, the key issue of the filter couple is the wavelength matching between the filter and the σ component. The center wavelength of the filter can be finely tuned by tilting the filter angle within a small range. The tuning range is limited to 8 degrees to prevent the collapse in filter bandwidth and to ensure a reasonable polarization fraction.
Another key issue is that the light delivered from the fiber cannot be coupled to the filter directly because the divergence illumination would cause serious widening. To cope with it, a filter coupler is developed. Figure 4 shows the structure of the filter coupler. It contains a collimation lens and a focusing lens, the filter is installed on a highly precise rotating stage (CONEX-AG-PR100P), and the cone light from the fiber is transformed to a parallel state by the collimation lens for ensuring the actual bandwidth is close to the minimum bandwidth. The minimum increment of the stage is 0.001 degree corresponding to nearly 0.0003 nm wavelength change of the filter, which further meets the requirements of MSE diagnostic.
The relationship between the filter peak transmission wavelength and the tilt angle needs to be calibrated before operation. To do so, a tungsten lamp illuminates the optical fiber that is connected to the filter coupler, a short fiber is mounted to the focusing lens and connected to a high resolution spectrometer [14], and the transmission spectra are shown in figure 5(a). The central wavelength and the FWHM are analyzed by Gaussian fitting. The variation of the center wavelength and the FWHM for one channel is presented in figures 5(b) and (c). When the stage angle is 90 degrees, the light incident is perpendicular to the filter. The relationship between the center wavelength and the tilt angle is of a quadratic shape. When the tilt range exceeds 8 degrees, the filter bandwidth will exceed 0.5 nm, which is significantly higher than the spectra broadening of the σ component and should be avoided at operation.
An integrated current output photoelectric multiplier tube (PMT H10721-20) of Hamamatsu Photonics Co. LTD is selected as the photon sensor and its response peak wavelength is around 630 nm. A self-developed high-precision current–voltage converter amplifier is applied for converting the PMT current signal (μA) to voltage signal (V), then, the magnified signals are connected to the phase-locked amplifier and the acquisition system. The frequency response of this amplifier is shown in figure 6, showing that the amplifier can work well within 100 kHz, which includes the major harmonics of the MSE signal.
The two-second harmonic component (84/94 kHz) signal is extracted by two-phase lock-in amplifier (MFLI-500 of Zurich Instruments). The reference signals are coming from the dual PEM controller. Two ADLINK PXI-2022 acquisition cards are used to provide 32-channel readout of the lock-in amplifier output signals with a sampling rate of up to 250 kHz per channel. This data acquisition system is part of the EAST data acquisition system and supports EAST long-pulse data acquisitions. All the MSE signals are stored in the MDS-plus server.
To demonstrate the performance of the MSE system, we analyze a typical EAST divertor discharge (No. 101148). Figure 7 shows the waveform of the discharge, Ip, βp, and li represent plasma current, poloidal beta, and internal inductance, respectively. The reconstructed result is given in figure 8. The reconstructed q profiles with (red) and without (blue) MSE signal constraints are compared at the time slice t = 7.9 s. The blue spots are the polarization angles measured by the MSE system, and the red line is the fitting result. When the MSE constraint is imposed, the qmin value is further reduced. This result is consistent with the expectation that the external magnetic data alone provides little or no information on the central q.
The quality of the current profile reconstruction is further verified by the location of q = 1 rational surface, which can be obtained experimentally from the inversion radius of the sawtooth oscillation by the ECE diagnostic. As shown in figure 9(a), the q = 1 surface is located at 2.025 m (low field side) at t = 7.9 s while the ECE suggests that the q = 1 surface is at R = 2.01 ± 0.03 m, which is in excellent agreement with the q profile reconstructed using MSE data.
A dual-PEM-based multi-channel MSE diagnostic system has been developed and commissioned in EAST. At present, only ten channels are equipment, which would be extended to 23 channels in the future. The polarization angles are deduced by the output of the phase lock-in amplifier. the preliminary result of a q-profile by equilibrium constraints using the measured pitch angle is obtained. The q(0) < 1 result is observed, and the position of the q = 1 surface is further verified with ECE sawtooth signal.
This work is supported by the National MCF Energy R & D Program of China (No. 2019YFE03040000), National Natural Science Foundation of China (Nos. 12075280 and 11805236), Anhui Provincial Natural Science Foundation (No. 1908085J01), and CAS President's International Fellowship Initiative (No. 2022VMB0007), Comprehensive Research Facility for Fusion Technology Program of China (No. 2018-000052-73-01-001228). Special thanks to Christopher Holcomb for his valuable advice on the development of MSE system on EAST.
[1] |
Levinton F M et al 1989 Phys. Rev. Lett. 63 2060 doi: 10.1103/PhysRevLett.63.2060
|
[2] |
Wróblewski D et al 1990 Rev. Sci. Instrum. 61 3552 doi: 10.1063/1.1141569
|
[3] |
Wróblewski D et al 1992 Rev. Sci. Instrum. 63 5140 doi: 10.1063/1.1143463
|
[4] |
Levinton F M 1992 Rev. Sci. Instrum. 63 5157 doi: 10.1063/1.1143466
|
[5] |
Stratton B C et al 1999 Rev. Sci. Instrum. 70 898 doi: 10.1063/1.1149318
|
[6] |
Fujita T et al 1997 Fusion Eng. Des. 34–35 289 doi: 10.1016/S0920-3796(96)00659-X
|
[7] |
Ko J et al 2016 Fusion Eng. Des. 109–111 742 doi: 10.1016/j.fusengdes.2016.02.008
|
[8] |
Bretz N et al 2001 Rev. Sci. Instrum. 72 1012 doi: 10.1063/1.1319609
|
[9] |
Chen W J et al 2010 Rev. Sci. Instrum. 89 126103 doi: 10.1063/1.5049613
|
[10] |
Conway N J et al 2010 Rev. Sci. Instrum. 81 10D738 doi: 10.1063/1.3494254
|
[11] |
Levinton F M and Yuh H 2008 Rev. Sci. Instrum. 79 10F522 doi: 10.1063/1.2968699
|
[12] |
Hu C D et al 2015 Plasma Sci. Technol. 17 817 doi: 10.1088/1009-0630/17/10/02
|
[13] |
Yu Q J et al 2020 Fusion Eng. Des. 153 111516 doi: 10.1016/j.fusengdes.2020.111516
|
[14] |
Li Y Y et al 2014 Rev. Sci. Instrum. 85 11E428 doi: 10.1063/1.4890408
|
[1] | Haochen FAN, Guoqiang LI, Jinping QIAN, Xuexi ZHANG, Xiaohe WU, Yuqi CHU, Xiang ZHU, Hui LIAN, Haiqing LIU, Bo LYU, Yifei JIN, Qing ZANG, Jia HUANG. Kinetic equilibrium reconstruction with internal safety factor profile constraints on EAST tokamak[J]. Plasma Science and Technology, 2024, 26(4): 045102. DOI: 10.1088/2058-6272/ad0d48 |
[2] | Hongbo FU, Huadong WANG, Mengyang ZHANG, Bian WU, Zhirong ZHANG. Effect of lens-to-sample distance on spatial uniformity and emission spectrum of flat-top laser-induced plasma[J]. Plasma Science and Technology, 2022, 24(8): 084005. DOI: 10.1088/2058-6272/ac6b8e |
[3] | Dan ZHANG (张丹), Anmin CHEN (陈安民), Qiuyun WANG (王秋云), Ying WANG (王莹), Suyu LI (李苏宇), Yuanfei JIANG (姜远飞), Mingxing JIN (金明星). Effect of lens focusing distance on laser-induced silicon plasmas at different sample temperatures[J]. Plasma Science and Technology, 2019, 21(3): 34009-034009. DOI: 10.1088/2058-6272/aaec9b |
[4] | Tao ZHANG (张涛), Haiqing LIU (刘海庆), Guoqiang LI (李国强), Long ZENG (曾龙), Yao YANG (杨曜), Tingfeng MING (明廷凤), Xiang GAO (高翔), Hui LIAN (连辉), Kai LI (李凯), Yong LIU (刘永), Yingying LI (李颖颖), Tonghui SHI (石同辉), Xiang HAN (韩翔), the EAST team. Experimental observation of reverse- sheared Alfvén eigenmodes (RSAEs) in ELMy H-mode plasma on the EAST tokamak[J]. Plasma Science and Technology, 2018, 20(11): 115101. DOI: 10.1088/2058-6272/aac9b5 |
[5] | Zhenhua JIANG (姜振华), Junfeng SHAO (邵俊峰), Tingfeng WANG (王挺峰), Jin GUO (郭劲), Dan ZHANG (张丹), Anmin CHEN (陈安民), Mingxing JIN (金明星). Effect of distances between lens and sample surface on laser-induced breakdown spectroscopy with spatial confinement[J]. Plasma Science and Technology, 2018, 20(8): 85503-085503. DOI: 10.1088/2058-6272/aabc5e |
[6] | Jia FU (符佳), Bo LYU (吕波), Haiqing LIU (刘海庆), Yingying LI (李颖颖), Dongmei LIU (刘冬梅), Yongqing WEI (魏永清), Chao FAN (范超), Yuejiang SHI (石跃江), Zhenwei WU (吴振伟), Baonian WAN (万宝年). Development of signal analysis method for the motional Stark effect diagnostic on EAST[J]. Plasma Science and Technology, 2017, 19(10): 104001. DOI: 10.1088/2058-6272/aa7941 |
[7] | Linghan WAN (万凌寒), Zhoujun YANG (杨州军), Ruobing ZHOU (周若冰), Xiaoming PAN (潘晓明), Chi ZHANG (张弛), Xianli XIE (谢先立), Bowen RUAN (阮博文). Design of Q-band FMCW reflectometry for electron density profile measurement on the Joint TEXT tokamak[J]. Plasma Science and Technology, 2017, 19(2): 25602-025602. DOI: 10.1088/2058-6272/19/2/025602 |
[8] | Shiheng YIN (尹诗衡), Li REN (任力), Yingjun WANG (王迎军). Plasma graft of poly(ethylene glycol) methyl ether methacrylate (PEGMA) on RGP lens surface for reducing protein adsorption[J]. Plasma Science and Technology, 2017, 19(1): 15501-015501. DOI: 10.1088/1009-0630/19/1/015501 |
[9] | QU Hao (屈浩), ZHANG Tao (张涛), ZHANG Shoubiao (张寿彪), WEN Fei (文斐), WANG Yumin (王嵎民), KONG Defeng (孔德峰), HAN Xiang (韩翔), YANG Yao (杨曜), GAO Yu (高宇), HUANG Canbin (黄灿斌), CAI Jianqing (蔡剑青), GAO Xiang (高翔), the EAST team. Q-Band X-Mode Reflectometry and Density Profile Reconstruction[J]. Plasma Science and Technology, 2015, 17(12): 985-990. DOI: 10.1088/1009-0630/17/12/01 |
[10] | XU Chao (许超), OU Yongsheng (欧勇盛), Eugenio SCHUSTER, and YU Xin(于欣). Computing Open-Loop Optimal Control of the q-Profile in Ramp-Up Tokamak Plasmas Using the Minimal-Surface Theory[J]. Plasma Science and Technology, 2013, 15(5): 403-410. DOI: 10.1088/1009-0630/15/5/02 |
1. | Liu, D.M., Sun, K.D., Chang, Z.Y. et al. FPGA-Based Digital Lock-In Technique for Real-Time Measurement of Polarization Angle in MSE Diagnostic on EAST. IEEE Transactions on Instrumentation and Measurement, 2025. DOI:10.1109/TIM.2025.3556195 |
2. | Qian, Z.H., Xiang, N., Huang, Y.H. et al. Equilibrium reconstruction constrained by the consistency of current simulation on EAST. Nuclear Fusion, 2024, 64(5): 056028. DOI:10.1088/1741-4326/ad35d7 |
Item | Value |
Clear aperture | 50 (mm) |
Focal length | 112 (mm) |
Objective distance | 1933 (mm) |
Object numerical aperture | 0.012 93 |
Image numerical aperture | 0.22 |
Magnification | 1/17.01 |